Solar Power: How to Effectively Harvest the Power of the Sun
11 minute read
Updated on: 14 Dec 2020
In 2 hours, the Sun hits the Earth with as much energy as we use worldwide in a year.
Let’s talk about light quickly before we dive into how solar panels turn it into useful energy.
What is light really?
We can think of light as a wave. The distance between the peaks of the wave is called its wavelength. Red light has a wavelength of around 700 nanometres (700 billionths of a metre) while blue light has a wavelength of around 450 nanometres
:
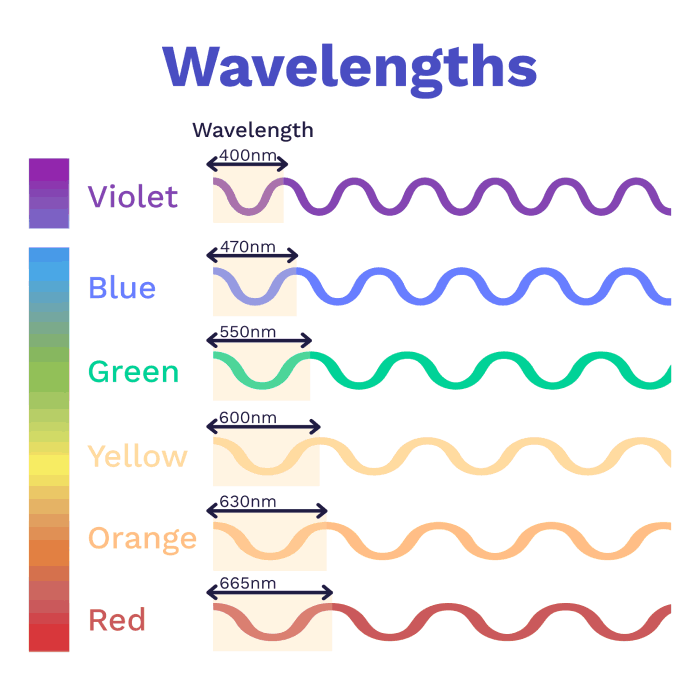
Wavelengths
The full set of wavelengths is called the Electromagnetic Spectrum. Only a small part of it is the visible light we can see:
Light carries energy - the shorter the wavelength, the more energy it carries.
Solar thermal is the most intuitive form of energy, so let’s start with that.
Solar thermal
On a sunny day you feel warmer in the sunshine than in the shade, right? This is because when your skin absorbs sunlight it turns part of it into thermal energy. We can use this heat!
Concentrated solar power (CSP) uses a large number of mirrors to concentrate sunlight onto a small area. The concentrated light is used to heat a fluid (water, oil, or molten salt) to very high temperatures. This heat can then either
- be used for heating,
- stored for later,
- or be converted to electricity by evaporating water and generating steam, which is used to spin a turbine. This works like most other power plants. Here’s the full process in one image:
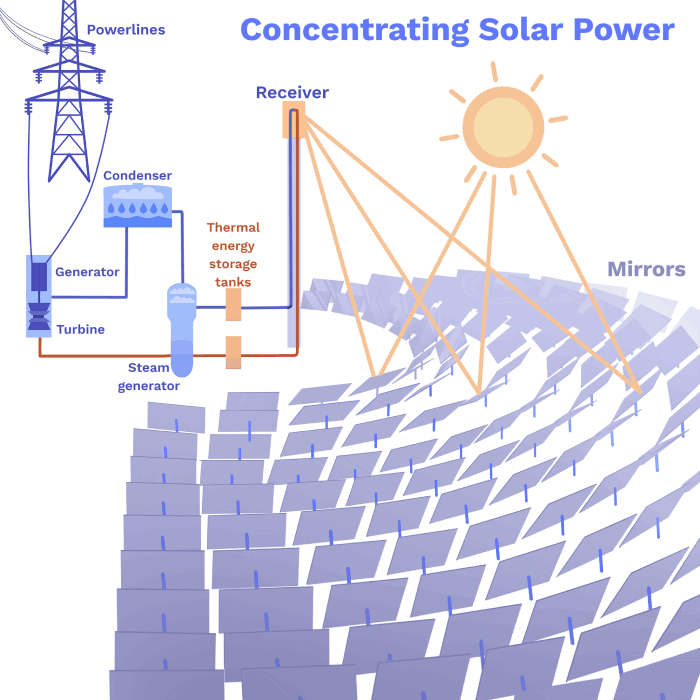
Concentrating Solar Power (CSP)
Today, electricity from CSP Solar Thermal is expensive, but it has some unique benefits
:
- It can be integrated with thermal storage to deliver electricity on demand.
- It contributes to power system stability and flexibility.
Solar thermal can also be used to heat homes. Solar thermal panels or solar thermal collectors absorb the sun’s light energy to warm up a fluid passing through the panel. This is then used to heat water that can be used for various domestic applications.
The reverse light bulb: turn light into electricity?
With Solar Photovoltaic (Solar PV) technology, we convert light directly into electricity. The average price of electricity generated this way is already similar to that of fossil fuels
- sounds promising! How does it work?
Most solar cells are made of silicon. Silicon has 4 electrons in its outer electron shell. When many silicon atoms come together, they form a crystalline lattice, due to what’s known in chemistry as covalent bonds. This would look as follows:
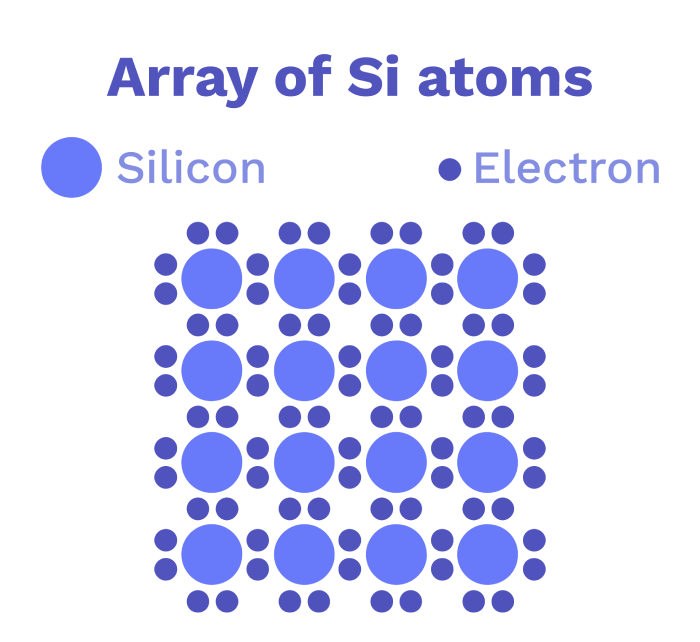
Silicon Structure
If we add a small number of atoms with 5 electrons in their outer shells (like phosphorus), the compound will still form crystals. However, there will be some free electrons floating around because they simply don’t fit anywhere in the crystal structure.
On the flipside, if we add an element with only 3 electrons in its outer shell (like boron), we’ll have an awkward electron hole in the structure.
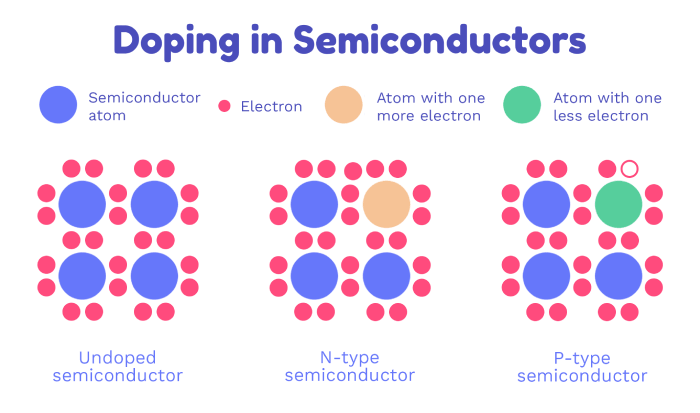
N-type and P-type Semiconductor
So what do we call these different materials?
- Silicon plus an element with 5 outer electrons is called an n-type semiconductor (n for negative). This is because Silicon usually has 4 outer electrons. With 5 outer electrons, it has an excess of electrons, making it negative.
- Silicon plus an element with 3 outer electrons is called p-type semiconductor (p for positive).
These names can be a bit misleading! Both n-type and p-type materials have a neutral charge (neither negative nor positive). So, why are they called positive and negative? Let’s look at the structures shown above. The n-type material has an extra negatively charged electron, while the p-type material has a positively-charged electron-free hole.
When a free electron from the n-type half makes it to the p-type half, it fills a hole there.
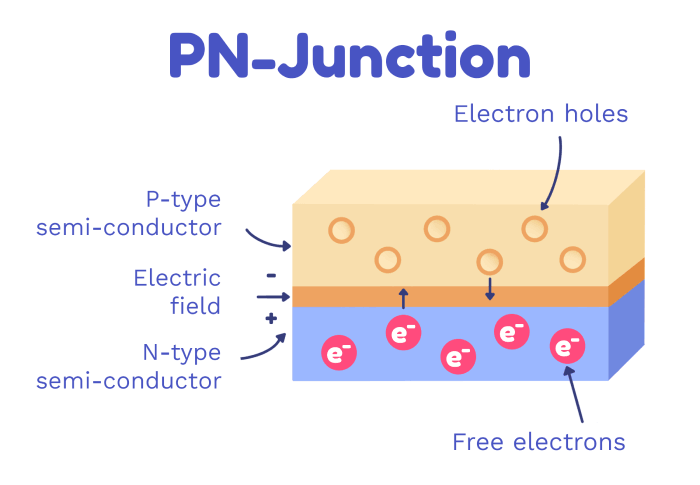
PN-Junction
The hole-filling happens in a small stripe where the p- and n-type halves touch. This is called the depletion region because this area has been “depleted” of holes.
This is fine for a bit. However, once many holes have been filled, the negative charge becomes strong. This produces what’s called an electric field barrier. At this point, electrons are pushed away from the depletion region by the electric field and no more holes are filled.
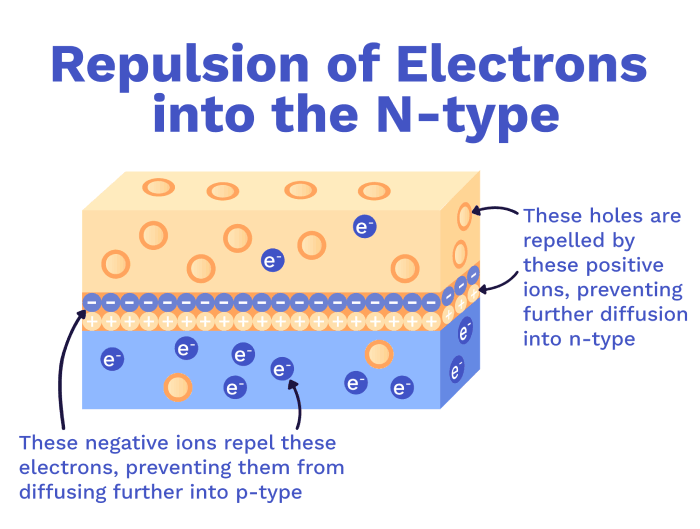
Repulsion of electrons into the n-type
Where does sunlight come into play?
Light carries energy. When light hits the depletion region, it can cause electrons to leave the holes they filled.
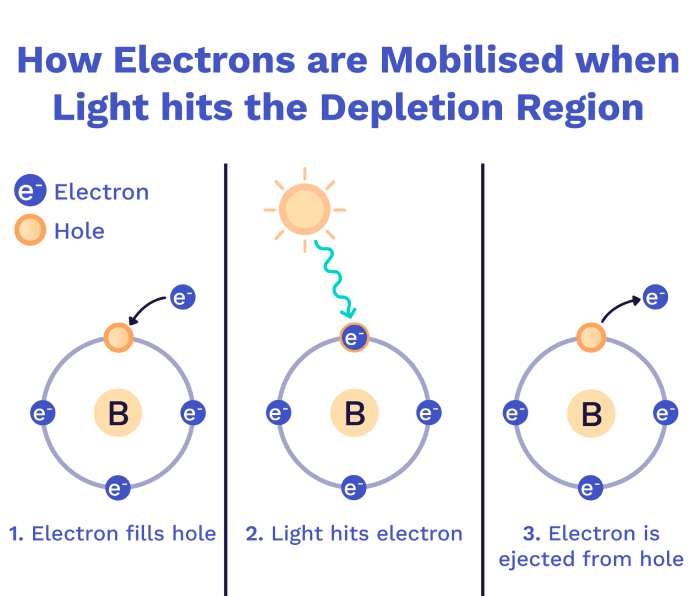
How electrons are mobilised when light hits the depletion region
The negative ions around the ejected electron repel it back into the n-type material. With one less electron filling a hole, there is (electromagnetic) room for a new one!
Electrons moving through a wire? That’s a current! And that’s how we get electricity using Solar PV.
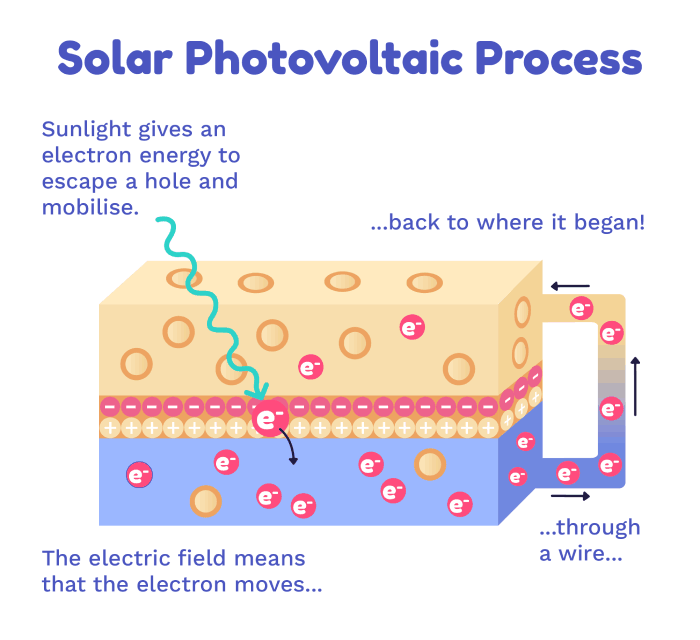
Solar Photovoltaic Process
When the electron is back at the p-type side, the solar cell goes back to its original state and the process can repeat for as long as the sun shines. Lovely!
In summary, the following cycle happens over and over again:
- An electron inside a hole is hit by light. The light’s energy causes the electron to be ejected.
- The electric field created by the ions in the depletion region pushes the electron towards the n-type side.
- Now there is an additional hole in the p-side region. This hole wants to be filled.
- An electron takes the backdoor through the wire or circuit to fill that space.
Is solar sustainable?
But what happens after those 25 to 30 years when the panels stop working? Sadly, that’s largely an open question. To prepare for when the solar panels built today become waste, we need to develop affordable large-scale recycling methods
.
How much land to produce all energy with Solar PV?
We’ll need three numbers to calculate a rough estimate:
- Humanity uses 157,000 TWh of energy per year
.
- The USA is hit with around 250MW of power per km² on average
.
- Modern solar PV panels operate at around 20% efficiency
, meaning they can turn 20% of the light energy that strikes them into electricity.
Due to the 20% efficiency, covering 1 km² with solar panels provides 50 MW of power. Remember that energy = power x time? So the amount of energy that is produced by these panels in one year will be 50 MW x 365 days x 24 hours = 438 GWh. This is equal to 0.438 TWh.
To cover the 157,000 TWh humanity uses, we’ll, therefore, need 157,000 / 0.438 = 358,500 km² of solar panels. Or in simpler terms, the area of Germany.
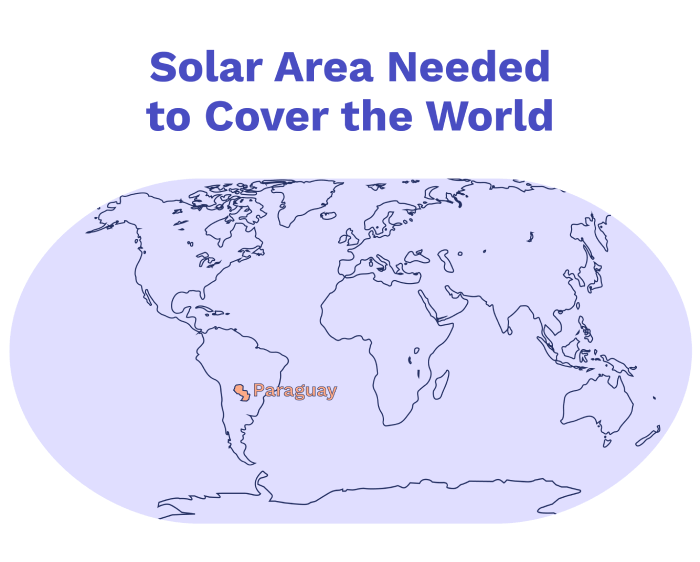
Solar area needed to supply the whole world with energy
That’s a lot, but not unreasonable. We cover 34% of the Earth’s land with crops and livestock for food, so a solar PV farm the size of Germany (0.3% of the Earth’s land
) is not a big deal in comparison.
However, deploying solar energy on an extremely large scale brings problems with it that are overlooked by the calculation above. The most prominent of these problems is that the supply of solar energy is very variable: we can’t make energy at night, but when the sun shines, we can make too much
! We’ll look at ways of solving this later.
Can we improve Solar PV and Solar Thermal?
There are many ways of doing Solar PV. Crystalline silicon PV (the technology we just discussed) absorbs around 20% of the solar energy that hits it. Other solar technologies show up to 40% efficiency, but at higher costs
.
So called ‘thin film’ techniques could become even cheaper and more environmentally friendly. To give you a sense of the development speed, look at this graph:
Concentrated solar power (CSP) systems could be deployed on a large scale today - we have the raw materials to build them. Although CSP is currently more expensive than fossil fuels it has the potential to become more viable when scaled up
.
A far less explored but still promising technology is Artificial Photosynthesis. If we can make this work at scale, it would solve many problems at once - more on that in the chapter on ‘Hydrogen’. Don’t skip ahead though! The chapters in between cover technologies that are just as exciting and important.